Given the level of investment required to get geothermal projects off the ground and see them through to completion, having a comprehensive and real-time view of a geothermal reservoir’s viability as early as possible is crucial.
But in the same way that the oil and gas industry deals with carbonate, basement and coalbed methane reservoirs, there are ways to model the subsurface approach to geothermal reservoirs – methods which allow us to better identify the projects presenting the biggest opportunities.
Reservoir modelling helps stakeholders build a robust business case, providing in-depth and live analysis of the drill site, its environmental make-up, how it might respond when work begins and, perhaps most importantly, its possibilities.
Needless to say, this insight is priceless to geothermal energy site developers and project stakeholders. Providing this level of understanding and confirming a reservoir’s commercial viability means geothermal energy developers can deliver successful projects and ultimately, bring sustainable geothermal energy sources onstream.
As experts in this rapidly growing space, we have set out our approach to geothermal reservoir modelling below, alongside some of the other established methods that are used to assess geothermal energy reservoirs.
Our approach to geothermal modelling
There are a number of techniques deployed to model geothermal reservoirs. At AGR, we employ the 3D and 4D static (or geological) and dynamic (or simulation) models, characterising the reservoir’s structural and property aspects to give us a comprehensive geological description of the energy reservoir and its dynamic behaviour over time. Constructing such models is a multidisciplinary effort between the fields of geophysics, geology, petrophysics, reservoir engineering and reservoir modelling.
The reservoir models for geothermal energy studies typically have an architecture signified by an integrated matrix-fractures duality. The matrix part of the model defines the main energy storage rock volume with its vital components and characteristics, whereas the fractures element represents the connectivity network, or transportation ‘highway’ for energy, with its physical signatures.
The modelling process includes gravimetric, magnetic, seismic data and well exploration and production data. These are analysed using both exploratory and confirmatory statistical methods and tools, often in labs.
What is a geothermal reservoir static model?
The static – or geological – 3D model is an integrated model containing the reservoir’s structural and property characteristics, expressed in a conglomerate of integrated sub-models.
The structural part comprises fault and fracture networks, geological formations, zones, segments and layering, and their specific attributes. The property part of the model includes various geological rock type ‘facies’ and expressions for their individual porosity, permeability, phase saturations, fracture intensity/density and aperture characteristics with specific attributes.
Static volume calculations using reservoir-specific energy and formation variables are performed, producing numeric and graphic estimates for aspects such as gross, net, pore, saturated and in-place energy volume figures, given a specific model realisation.
Alongside this, uncertainty and sensitivity analyses are also conducted, producing several stochastic model realisations using Monte Carlo algorithms. The output could be uncertainty ranges – such as P10-P50-P90 volumetric figures – or Tornado diagrams, to understand which aspects of the reservoir are more or less critical. This analysis guides and supports decision-making processes relating to reservoir development and management.
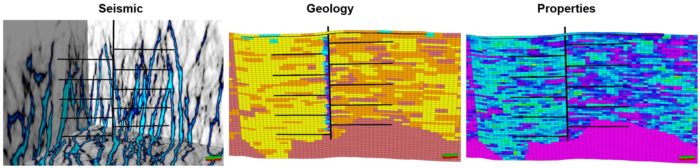
The resulting 3D geothermal reservoir static model, with its various property sub-models for the matrix-fractures duality, is used as a starting point for the dynamic modelling process.
Expanding on the dynamic geothermal reservoir model
To produce the dynamic geothermal reservoir model, we fit additional physical parameters to the static model, such as temperature and pressure fields, plus various dynamic aspects, financial guidelines, as well as constraints from reservoir engineering and production technology. The latter could be various layouts for the well design – or altogether different means for stimulating the reservoir, such as ‘Fish Bone’ and ‘Manara’ technologies.
As touched on earlier, the intention of the dynamic model is to evaluate the reservoir’s dynamic behaviour over time, optimising reservoir development and management. Geothermal dynamic reservoir modelling helps to maximise production, ensure safety and unlock new efficiencies all while minimising cost, risk and environmental impact.
There’s more to it, though. The dynamic model can also incorporate a historical time series of production data, if available. Then, history match simulation results can be used in a feedback loop to update and fine tune the vital parameters of the static model. This approach also improves the accuracy of forecasts around future geothermal reservoir performance and geothermal energy production, with greater precision and reliability in the model predictions.
The static and dynamic geothermal reservoir models are also used for planning infill well drilling campaigns, to optimise energy drainage throughout the reservoir’s lifecycle.
Overview of alternative geothermal reservoir modelling approaches
We’ve outlined our approach above, but various methods of geothermal reservoir modelling have emerged and evolved over the years. Different approaches are often combined and – depending on the unique characteristics of a given geothermal reservoir – these can be categorised as follows: